Biology as design is transforming how we live, work, and learn. K-12 science education must catch up.
In recent years, biotechnologies have begun to move out of laboratories and into daily life in astonishing ways. For example, the Arctic apple, a genetically modified fruit that doesn’t turn brown when bruised or sliced, is now available in supermarkets across the country. Or consider CAR T-cell therapy, which has allowed hundreds of people with cancer to be treated successfully using their own altered immune cells. These bioengineered cells appear to be more effective than drugs or radiation at targeting cancer cells for elimination.
The Arctic apple, CAR T-cell therapy, and many other innovations speak to a remarkable paradigm shift in biology, from merely observing nature to actively designing or redesigning it. Thanks to advances in the decoding and editing of genes, it has become possible to recode DNA and change how cells behave. And as the current COVID-19 pandemic makes clear, the need for these approaches is urgent: Today, scientists around the world are relying on these advances in gene editing to design a vaccine to defend against a threat that humankind has not experienced in recent history.
However, despite the significance of this paradigm shift for scientific, industrial, and commercial applications, it has barely made a ripple in elementary and even secondary life science classrooms. Our K-12 science standards and frameworks do not yet address these new biotechnologies. To date, even instruction in the basic principles of genetics — which are foundational to all biotechnologies — has been extremely limited. Of those few students who do have opportunities to learn about genetics, and whose schools have newer lab equipment, some are taught how to extract DNA. But even those students are rarely given chances to experiment with genetic material and make something new — that is, to biodesign.
To be clear, we are not talking about giving students chances to create Frankenstein monsters, but only to learn, in a hands-on way, what it means to practice this emerging branch of science and, in turn, to reflect on and discuss its larger implications. It is about giving students a chance to engage these fields not only as an occupational endeavor, but also a civic one, in which learners are able to make thoughtful judgments and decisions about the field and its myriad impacts. Like many new scientific advances, biodesign raises complex moral dilemmas that are relevant even to students who are not themselves planning to make a career in the sciences. Consider, for example, the recent controversy surrounding the Chinese researcher who edited the genome of twins still in the embryo stage so that their white blood cells might later be resistant to HIV. Many people were outraged in response, arguing that scientists have no right to experiment with human life in this way. No doubt, such debates over how to distinguish between ethical and unethical uses of this technology will become increasingly common in the coming years, and in order to perform their roles as citizens, our students will need to understand what is being argued.
Like many new scientific advances, biodesign raises complex moral dilemmas that are relevant even to students who are not themselves planning to make a career in the sciences.
A new science built on old ideas
Although the biotechnologies that allow for gene editing are new, the basic concept behind biodesign goes back centuries — humans have always taken steps to shape nature’s evolutionary trajectory, from the grafting of plants to the breeding of dogs and so on. But until inventions like CRISPR (a gene-editing tool developed by Jennifer Doudna and Emmanuelle Charpentier in the 2000s), actively editing DNA was a messy and unpredictable enterprise. CRISPR made it a controlled and repeatable action by providing biotechnologists with a programmable scalpel that could cut DNA at a precise location to make changes. Combined with advances in synthetic biology, this has enabled scientists to reprogram cells so that they function in altogether new ways.
Unlike many biotechnologies that leverage our understanding of DNA, synthetic biology focuses on generating new practical and usable products never before seen in nature. And keep in mind that new kinds of apples, cancer treatments, and vaccines are just the beginning. This approach has also been used to enrich yeast cells with vitamins to enhance crop yields and improve food security. In the coming years, scientists may be able to genetically alter cells to produce pigments, creating sustainable and less toxic methods of printing and dyeing. Or they may harness photosynthetic algae to generate clean electricity. For humankind, the potential advancements (and, yes, the possible dangers) are endless, which only underscores the need to update the K-12 science curriculum.
Opportunities and challenges
The Next Generation Science Standards (NGSS) represent a much-needed effort to modernize STEM education to reflect the ways in which experienced scientists draw on concepts, skills, and practices from various disciplines. For example, partnerships among engineers and life scientists have been common for many years already, since long before the advent of biodesign. And as the BioBricks Foundation (an organization of scientists and engineers who work on bioengineering for the public good) has demonstrated, engineering genetic material is strikingly similar to computer programming. Further, research into the everyday work of biologists, computer scientists, physicists, and other STEM professionals has made it clear that they often look for ways to learn from each other and bridge their fields. It’s time to bring these connections into the classroom.
Of course, this raises a pressing challenge for teachers: How can they equip their students with a basic grasp of a new scientific field — biodesign — that they, themselves, did not learn about in school, and which they may not fully understand? Clearly, we need more opportunities for teachers and students alike to learn about and do hands-on work with biotechnologies. Such opportunities were once mostly limited to the university level, but the development of low-cost, portable lab devices has made it possible for learners of all ages to design with biology, practically anywhere. For instance, middle and high school students can use the BioMaker Lab developed by the University of Pennsylvania Graduate School of Education to genetically modify and grow bacteria cells so that they glow in the presence of a particular substance. Students can then use these bacteria in a biosensor that detects environmental pollutants.
And for high school science teachers who want to use synthetic biology in their classrooms, the BioBuilder Foundation offers a promising model for professional development. The foundation’s multiday trainings, often co-led by educators and scientists, are held in cities across the United States, showing teachers how to carry out a full catalogue of commercially available lab activities designed for high school students. For example, in the Eau that Smell lab, teachers and students observe firsthand how synthetic biology can be leveraged to produce synthetic aromatics or flavor food additives when they genetically modify bacteria to selectively emit a banana scent at different stages of cell growth. No doubt, more such efforts will be needed to make a meaningful impact on the practitioner pipeline, but the program offers a promising example of how to help teachers get up to speed on new scientific breakthroughs.
At the same time, teachers will also need to be supported in creating lessons and units that address the ethical questions raised by these biotechnologies (Gutmann, 2011). For instance, creating reproductively sterile strains in male mosquito species could reduce the spread of endemic viruses like the flu or COVID or parasitic-induced diseases like malaria, but it could also disrupt food webs that are crucial to a whole network of organisms, including humans. How should such risks and rewards be weighed, and who should decide which risks are worth taking?
We know that middle and high school students can easily be engaged in debating ethical issues, but how much and what sorts of content knowledge will they need to make informed arguments about such cutting-edge technologies? To help learners develop critical literacies on these topics, we’ll need research into how young people construct knowledge about the field, how they critically examine that knowledge, and how to support their ability to develop well-formed and decisive arguments about the complex and often messy issues these fields encompass. Policy makers, teachers, and researchers in education must all be part of the effort to determine how these and other new advances ought to shape science standards, curriculum, and teaching.
A new era in science education
Scientific literacy in K-12 education is a “means for students to assert and defend claims in science, demonstrate what they know about a concept, and convey what they have experienced, imagined, thought, and learned” (NGSS Lead States, 2013, p. xxi). Biology as design enables students to see scientific knowledge as a valuable resource for not only asserting and defending claims but also for designing applications that are of personal, economic, and critical societal relevance, from creating new colors, toys, and games to inventing new kinds of insulin, batteries, sensors, and so much more (Kafai & Walker, 2020).
In 2019, 37 student teams took part in the Biodesign Challenge. This was the first year high school students participated, and they wasted no time in making their mark. A high school team of three girls created a biodesign toy kit to introduce younger audiences to synthetic biology materials, including packaging made from mushrooms and a microbial-based plastic. The project, which earned a first runner-up prize, also called attention to the perils of plastic waste in the toy industry and the value of sustainable manufacturing. But what was especially exciting to us was that these K-12 students were making biodesign accessible to younger K-12 learners. The era of biology as design has arrived, and as more students learn about this science and become scientists, teachers, and citizens, they’ll be able to pass their knowledge on to future generations.
References
Gutmann, A. (2011). The ethics of synthetic biology: Guiding principles for emerging technologies. The Hastings Center Report, 41 (4), 17-22.
Kafai, Y.B. & Walker, J.T. (2020). Twenty things to make with biology. In Proceedings of Constructionism 2020 conference. Dublin, Ireland.
NGSS Lead States. (2013). Next generation science standards: For states, by states. Washington, DC: National Academies Press.
ABOUT THE AUTHORS
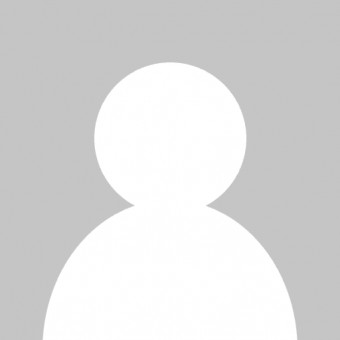
Justice T. Walker
Justice T. Walker is a postdoctoral research fellow at the University of Pennsylvania Graduate School of Education, in Philadelphia.
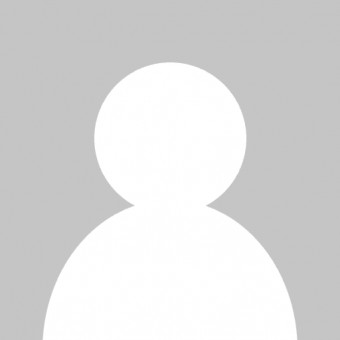
Yasmin B. Kafai
Yasmin B. Kafai is the Lori and Michael Milken President’s Distinguished Professor, at the University of Pennsylvania Graduate School of Education, in Philadelphia. Kafai is a co-editor of Designing Constructionist Futures. She and Quinn Burke are authors of the Connected Code.